The research by PhD candidate HU Jialiang Hu, Prof. LIN Jun from Yunnan Observatories (YNAO), and their co-authors has been published in Research in Astronomy and Astrophysics. The study focuses on the Quasi-Periodic Fast Propagating (QFP) wave trains in the early stages of solar eruptions, conducting a comprehensive and in-depth exploration of their composition and anisotropic characteristics.
Using the Titov-Démoulin model, the researchers conducted 3-dimensional (3D) numerical experiments to study EUV wavefronts (specifically QFP wave trains) during solar eruptions. During the upward eruption of a magnetic flux rope, wave phenomena emerge, exhibiting the wavefront expanding outwards in a dome-shaped manner, where the radial speed surpasses the lateral speed. This highlights the flux rope's upward motion in shaping the wavefront's 3D structure, which becomes more complex as it propagates.
The simulation reveals a striking phenomenon: a wavefront in the solar atmosphere can be divided into three regions. Region 1, known as the compression region, is characterized by a velocity divergence of less than 0 and constitutes the wavefront's main component. It comprises fast-mode shock wave that locally heat plasma. Region 3, the expansion region, with a velocity divergence greater than 0, is located on the rope's sides and composed of expansion waves cooling the plasma. Region 2, the transition region with zero divergence, lies between the first two regions and is the narrowest. This is attributed to the flux rope acting as a 3D piston, forming shock waves ahead and expansion waves behind.
To explore the origins of QFP wave, the researchers calculated velocity divergence at different times. The results indicate that the flux rope acts as an imperfect waveguide, with QFP waves originating from internal disturbances that bounce within it. These disturbances penetrate the flux rope's boundary into the corona, forming observed QFP waves. The wavefront speed (1,282 km/s) aligns with EUV wave speeds in high-resolution AIA data. Simulations show second and third wavefronts emerging at t=36 and t=72 seconds, suggesting a QFP wave period of approximately 36 seconds.
Using simulation data, the researchers synthesized EUV images, with characteristics resembling actual events, especially in the 193 and 211Å wavebands responsive to coronal fluctuations. The 94Å waveband response is weaker. EUV responses concentrate in the fast-mode shock wave region, impacting observed EUV wave layers: some perspectives capture full wavefronts from lower to upper atmospheres, while others capture only high coronal signals. The observed EUV morphology depends on shock wave distribution and observation perspective.
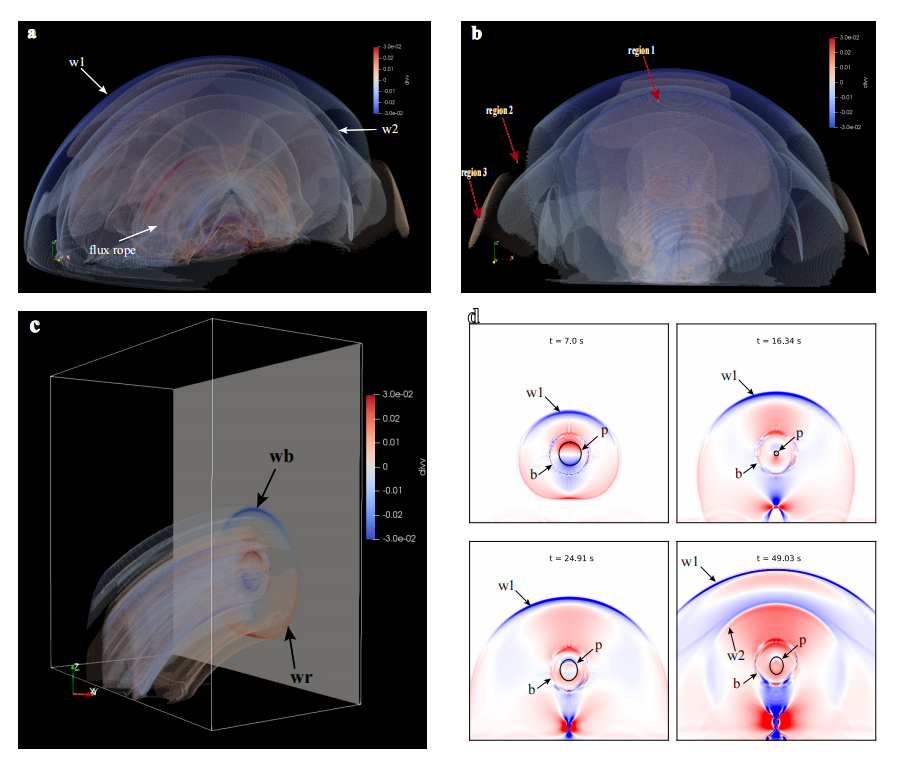
Figure 1: Analysis of the three-dimensional propagation process and mechanism of QFP waves. "b" represents the boundary of the flux rope, and "p" represents the disturbance within the flux rope. Image by HU.
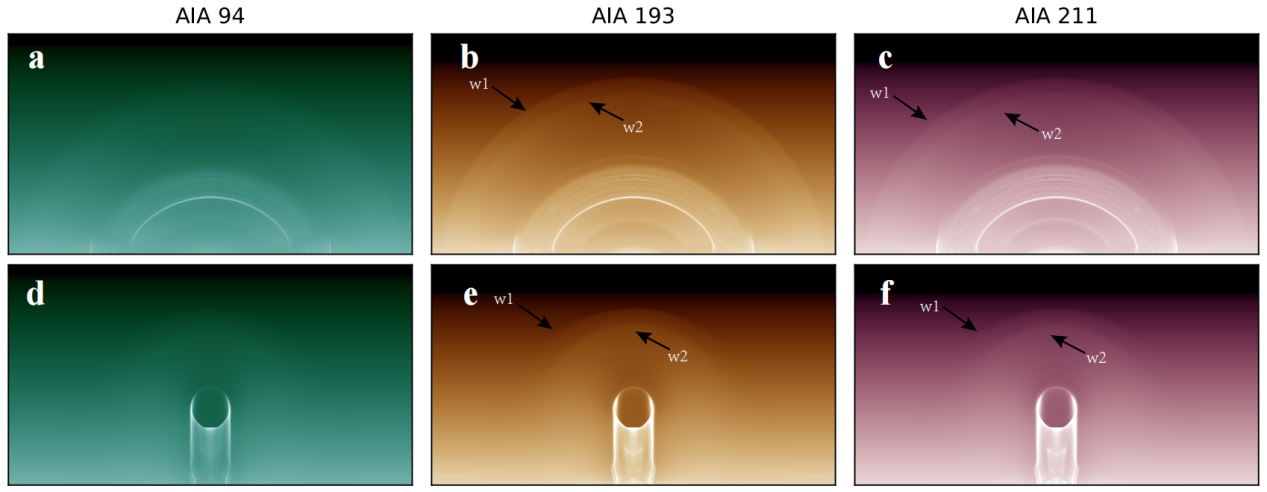
Figure 2: The synthesized images of AIA 94, 193, and 211 Å at t = 70 s. Image by HU.
Contact:
HU Jialiang
Yunnan Observatories, CAS
E-mail: hujialiang@ynao.ac.cn